
Using human iPSCs, it is theoretically possible to create tissues or organs with patient-derived cells, thus eliminating immunogenicity issues. These cells exhibit both transcriptional and epigenetic signatures similar to those of embryonic stem cells (ESCs), and thus they open up exciting scenarios for tissue engineering. Induced pluripotent stem cells (iPSCs) were generated for the first time in 2006 by Shinya Yamanaka 5 and marked a crucial milestone in the field of biomedical sciences. This is thus the objective of skeletal muscle tissue engineering, which aims to harness the knowledge derived from studying embryogenesis processes, and partly reproducing them in vitro.

To obtain such muscle grafts in vitro, appropriate myogenic precursors in a three-dimensional (3D) construct need to be engineered, pushing their differentiation to match the morphological and functional features of the native human muscle tissue. Fully-functional muscle grafts could also be exploited in lab-on-a-chip platforms for testing the efficacy, toxicity, and possible side effects of drugs, thereby significantly reducing (ideally eliminating) the need for animal sacrifices 4. In such cases, having healthy patient-specific muscle grafts developed in vitro and ready to be implanted in the impaired area would be highly desirable to restore tissue functionality. However, the skeletal muscle’s ability to self-repair may be impaired due to aging, genetic diseases 2, or injuries with volumetric muscle loss (VML) 3. MuSCs then fuse to form terminally differentiated multinucleated myofibers, thereby restoring the pool of highly specialized cells in the tissue 1. MuSCs are located between the cell membrane and the basal lamina of myofibers, and their activation leads to cell proliferation and eventually exit at G1 phase. In vivo, skeletal muscles can self-regenerate: after traumas or other tissue damage, resident muscle stem cells named muscle satellite cells (MuSCs), are activated.
Muscle synergy .05 series#
Skeletal muscles enable voluntary movements and, consequently, a series of dynamic interactions between individuals and their surrounding environment. The embryonic development process and the pro-myogenic role of the muscle-resident cell populations in co-cultures are also described, highlighting the possible clinical applications of iPSCs in the skeletal muscle tissue engineering field. Third, technologies to provide cells with biophysical stimuli, biomaterial cues, and biofabrication strategies are discussed in terms of recreating a biomimetic environment and thus helping to engineer a myogenic phenotype.
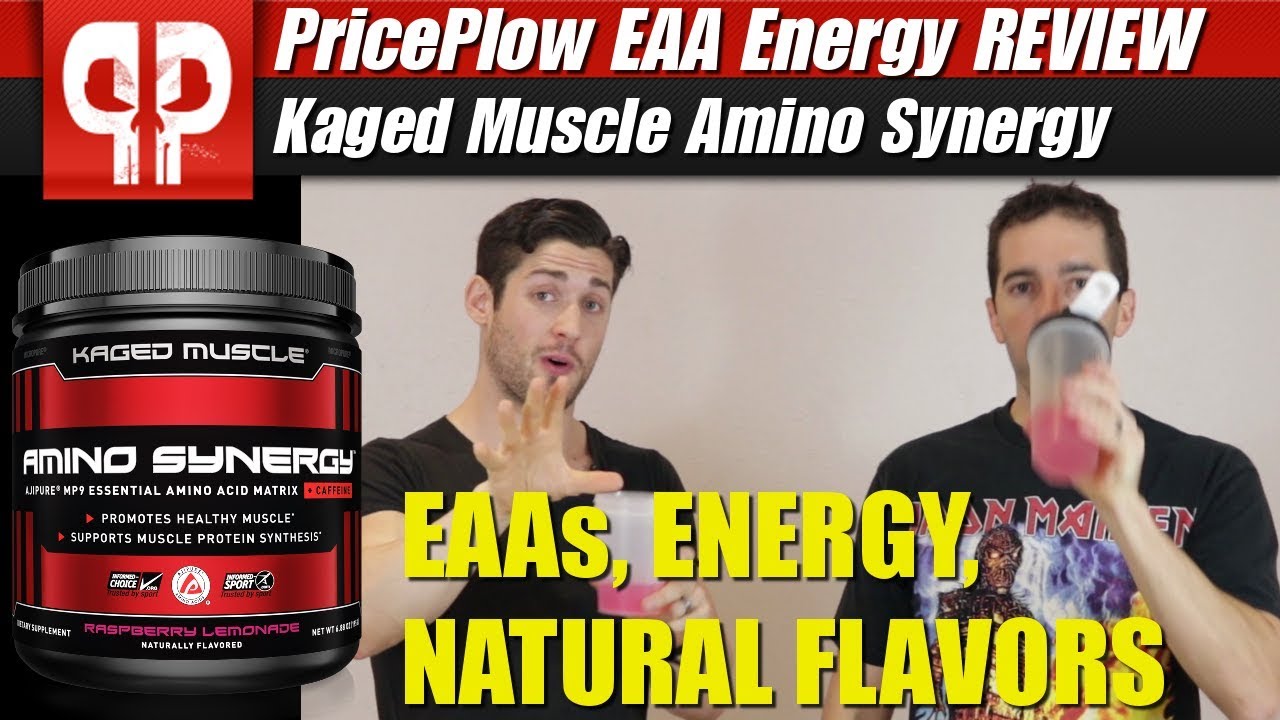
Second, transgenic and non-transgenic approaches for the myogenic induction of human iPSCs are compared. First, the overall organization and the key steps in the myogenic process occurring in vivo are described.

This review highlights the potential of this combination aimed at pushing the boundaries of skeletal muscle tissue engineering. Induced pluripotent stem cells (iPSCs) can generate myogenic progenitors, but their use in combination with bioengineering strategies to modulate their phenotype has not been sufficiently investigated. Although skeletal muscle repairs itself following small injuries, genetic diseases or severe damages may hamper its ability to do so.
